The shape-shifting superhero: Dictyostelium discoideum
Issue: Real superheroes
25 February 2014 article
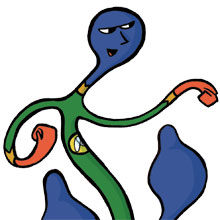
Dictyostelium discoideum is one of the social amoebae, a group of fascinating eukaryotic microbes with superhero, shape-shifting qualities, which is able to switch between a unicellular and a multicellular existence.
A hint at the developmental plasticity of social amoebae is the traditional classification according to the morphology of their multicellular fruiting bodies. The genus Dictyostelium typically develops unbranched fruiting bodies, so the name is derived from stelium (tower), along with dicty (net-like), these being phases of the multicellular and unicellular life habits, respectively. The archaic name for these amoebae is ‘slime mould’, a term that provokes the same grimaces in the Dicty research community as seen in cyanobacteria researchers when they hear the words ‘blue-green algae’. Dictyosteliida form a branch of eukaryotes separate from the fungi, and from plants and animals. Lacking a cell wall, they do resemble animal cells in organisation, except for the presence of a contractile vacuole, and many features of Dictyostelium make the genus a useful eukaryotic model in molecular biological and biomedical research.
FIG. 1. UNICELLULAR DICTYOSTELIUM
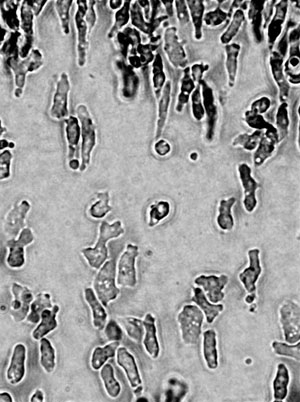
Dicty was, glamorously, first studied in the 19th century in horse dung. In its unicellular phase, motile, chemotactic cells (Fig. 1) phagocytose bacteria in animal dung, leaf litter or the top layers of soil rich in decaying organic material. Today, we grow Dicty on bacterial lawns in Petri dishes where plaques become visible after Dicty graze the bacteria off the agar surface – the wet leafy smell of Dicty cultures persists when it is grown on agar and is considerably more pleasant than the odour of Escherichia coli grown in the incubator. Early studies of Dictyostelium therefore provided an example of both a new phenomenon – phagocytosis – and a multicellular development process that kicks in when organisms become starved of their prey. The phagocytosing vegetative phase is followed by aggregation of the unicellular individuals – the ‘social’ phase (Fig. 2). First, a tower of cells forms, which tips over to form a pseudoplasmodium or ‘slug’ (Fig. 3), which then develops into a fruiting body standing 1–2 mm tall. This structure, described as looking like ‘a ping-pong ball balanced on top of a floppy wire’, is easily visible to the naked eye on agar plates (Fig. 4) – part of the charm of these organisms. Spore cells from the fruiting body have a tough cell wall, endowing resistance to temperature extremes, desiccation and digestion, and are dispersed ready to start a new life cycle when conditions are correct. These major morphological changes (Fig. 5) take about a day, and are accompanied by enormous changes in gene transcription and protein expression.
FIG. 2. AGGREGATING CELLS
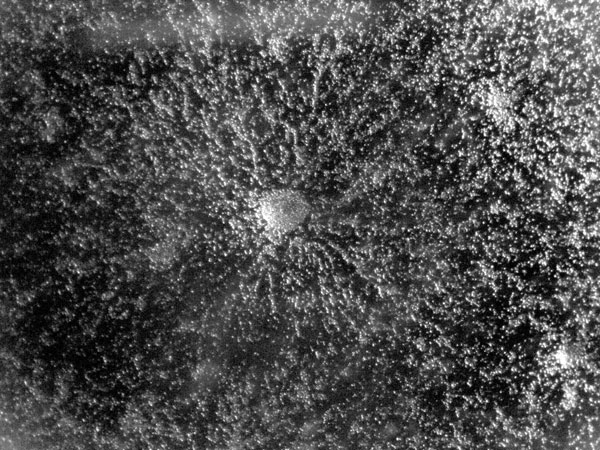
Klebsiella is a standard prey bacterium in the lab, but Dictyostelium can engulf members of many other genera. Indeed, it has been proposed that bacterial pathogenicity evolved to resist this kind of predatory amoeba. Thus, Dictyostelium can be a useful model host for several pathogens, including Mycobacterium species and Legionella pneumophila.
FIG. 3. SLUGS
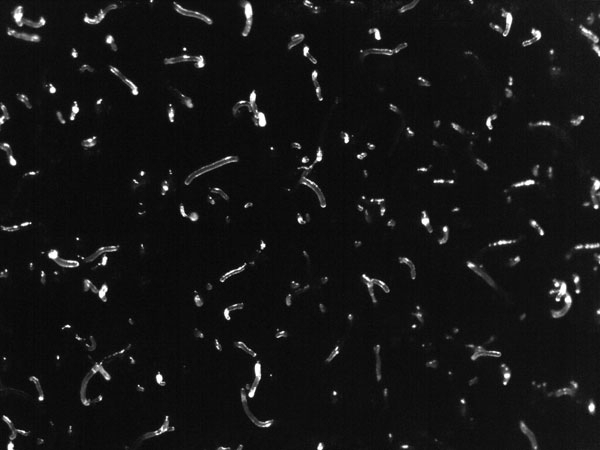
Another biological paradigm and interesting digression is the primitive farming behaviour of these amoebae described by Brock and colleagues in Nature in 2011. A form of symbiosis occurs between Dicty and its bacterial prey in which a proportion of colonies from environmental isolates of Dictyostelium seem to engage in bacterial husbandry, in which some prey bacteria are maintained within the Dicty fruiting bodies. This allows the seeding of the food source at a new location ready for the next generation.
FIG. 4. THE PING-PONG BALL SORUS ON THE FRUITING BODY STALK
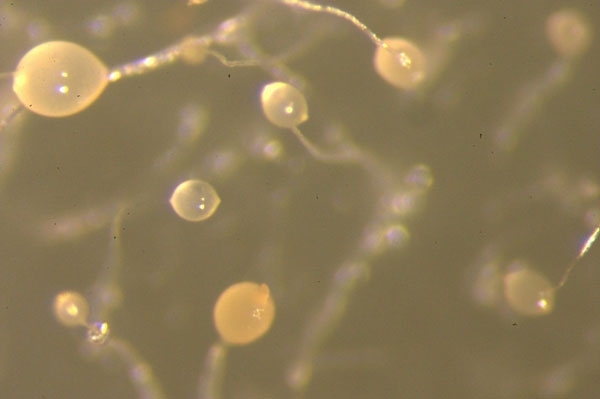
Astonishingly, the multicellular slug that forms from aggregated individual cells can be motile. The slug (Fig. 3) is approximately 2–4 mm long, is generally composed of 104–105 cells, and moves (forwards only) towards attractants such as light, heat and humidity, leaving behind a slimy trail from its extracellular cellulose matrix. This relocation in response to the environment allows formation of the raised fruiting body stalk and spore dispersal in a more favourable location. Chemotaxis is key to aggregation and culmination in this social phase, when approximately 100,000 cells signal to each other. Waves of regulated production and secretion of the chemoattractant cAMP are balanced by a degradation of cAMP using a constitutively expressed phospodiesterase enzyme. Wave-emitting centres develop and become aggregation centres; the extracellular cAMP induces ‘aggregation waves’ of neighbouring cells moving towards each other (Fig. 2, showing dendritic pattern), until a tightly aggregated mound is formed (Fig. 5). In these first steps, Dicty’s route to multicellularity differs from metazoan embryogenesis, when a complex organism is formed from a single starting cell via cell division. The cell diversity that develops in the fruiting body, however, makes this organism an excellent model for studying cell differentiation. Indeed, many processes of development in both Dictyostelium and metazoans are evolutionarily conserved, including communication between cells, differential cell sorting, pattern formation, stimulus-induced gene expression, and cell-type regulation.
FIG. 5. AGGREGATING MOUNDS
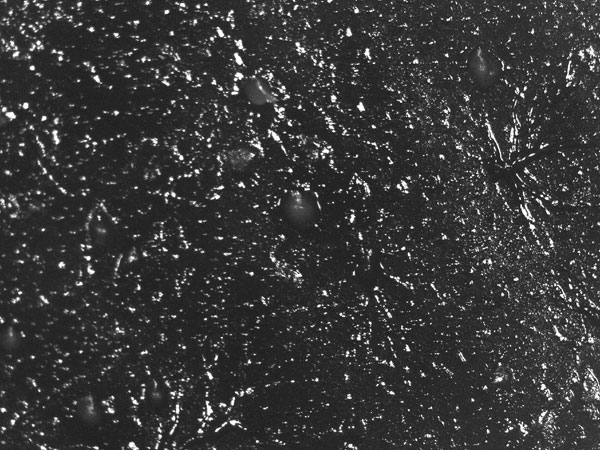
The cAMP periodic stimulus allows coordination of developmental gene expression, with different sensitivities to chemoattractant in different cell types. The fruiting body’s stalk cells are differentiated and distinct from the anterior (actively leading) part of the slug, whereas spores originate in the posterior. Via chemotactic cell sorting, mixed cells form chimeric organisms, which can be observed using fluorescently labelled cells either in wild-type or in mutants with aberrant cell-sorting phenotypes (Fig. 6). Notably, Dicty stalk cells perish during fruiting-body maturation, whereas the sorus is a ball of viable, haploid cells in spores that are ready for germination. This sacrifice of the stalk cells represents a large loss of culminating Dicty cells since the anterior’s prestalk cells represent 10–30% of the population. Meanwhile, prespore cells and a population called ‘anterior-like’ cells are intermingled in the posterior (Fig. 6). Prestalk cells and prespore cells therefore switch positions: anterior-like cells ultimately form the bottom of the fruiting body and the caps of the spores, with cells from the slug posterior on the top, forming the spores of the fruiting body. Via this quite remarkable transformation, Dictyostelium amoebae individuals altruistically join ranks to form a new, truly multicellular organism. Even more remarkably, not all the amoebae survive, cooperating by sacrifice to help a new generation start a new life elsewhere. Beyond the scope of this article are the cheaters – mutant strains or wild isolates of Dicty that do not respond correctly to the signal to become a self-sacrificing stalk cell.
FIG. 6. FLOURESCENTLY LABELLED RED CELLS WILL BECOME STALK, AND GREEN ONES SPORE CELLS
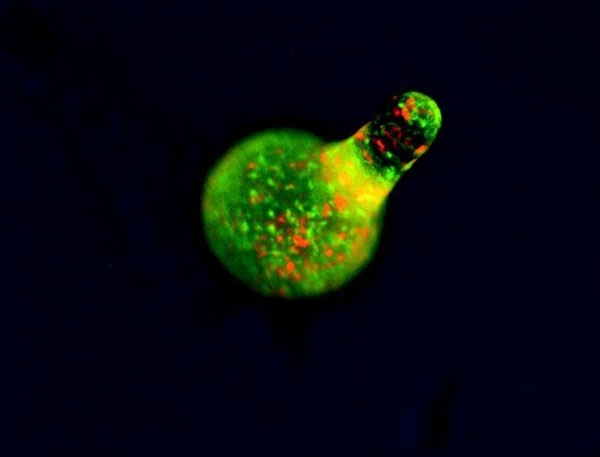
Unicellular Dictyostelium is irregularly shaped (Figs 1 & 7) as it moves through its environment; phagocytosing prey and responding to cAMP, it produces actin-rich pseudopods at the front of the cell and uses myosin to contract the rear. Consequently, Dictyostelium is also an important model organism for studying actin cytoskeleton dynamics, including cell–substrate adhesion and endocytosis, polarised cell growth and filopodia (finger-like extensions of the cell surface). The importance of actin is highlighted by a mutation in an actin-regulatory gene resulting in a Dicty that is unable to produce fruiting bodies. Dicty is not limited to the amoeboid type of motility (also seen in animal neutrophils and tumour cells): it can alternatively move via blebbing or with a single, flat, actin-rich lamellipod extending in the direction of movement.
A final morphological possibility for Dictyostelium is apparently a rare occurrence in the laboratory. In the sexual life cycle, two haploid amoeba cells of different mating types that meet in a moist and dark environment (lights off, please) can fuse during aggregation and form a giant cell nearly ten times the diameter or length of Dicty vegetative cells. This engulfs neighbouring cells by phagocytosis, forming a heterocyst with a thick exterior cell wall. Following meiosis in the heterocyst, a new generation of genetically distinct vegetative amoeba are released to follow a unicellular life.
FIG. 7. CYTOKINESIS IN DICTYSTELIUM
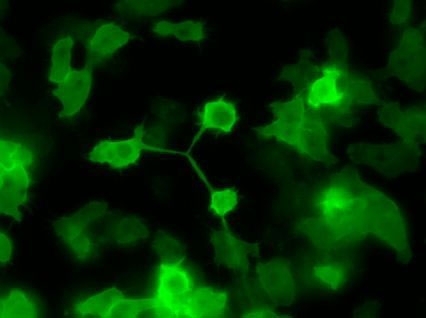
The Dicty life cycle as a whole is easily observed without magnification, occurs at room temperature, is fascinating, and relatively rapid. Cells are quite transparent and are amenable to imaging techniques, the genome was sequenced early (2005), and it can be transformed. Dicty’s limited cell types and known patterns of behaviour can be studied at the level of the organism, cell or molecular network. In addition, the superhero qualities of Dictyostelium have made it one of the model organisms chosen by the United States National Institutes of Health as part of its Model Organism Initiative and, indeed, it has become a tool for widely varying fields, from ecology and social evolution to biomedical science.
MEHAK RAFIQ & ELINOR THOMPSON
School of Science, University of Greenwich, Chatham Maritime, Kent ME4 4TB, UK
[email protected], [email protected]
FURTHER READING
Brock, D. A. & others (2011). Primitive agriculture in a social amoeba. Nature 469, 393–396.
Duleh, S. N. & others (2006). Morphological and functional analysis of Rac1B in Dictyostelium discoideum. Microscopy 54, 519–528.
Gonzalez-Kristeller, D. C. & others (2008). The P450 oxidoreductase, RedA, controls development beyond the mound stage in Dictyostelium discoideum. BMC Dev Biol 8, 8.
Santorelli, L. A. & others (2013). A new social gene in Dictyostelium discoideum, chtB. BMC Evol Biol 13, 4.
Williams, R. & others (2006). Towards a molecular understanding of human diseases using Dictyostelium discoideum. Trends Mol Med 12, 415–424.
Image: Figs. 1, 2, 3 and 5 M. Rafiq Fig. 4 E. Thompson Fig. 6. C. Thompson, University of Manchester Fig. 7 R Williams, Royal Hollway University of London. Illustrations by James B. W. Ilustration.