Understanding bacteria and challenges in microbiology
Issue: Why Microbiology Matters
05 May 2020 article
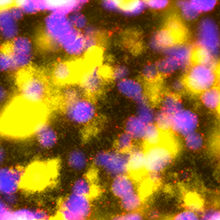
Bacteria are found in every habitat on Earth and there are approximately 10 times as many bacterial cells as human cells in the human body. This section highlights some of the many challenges we face understanding bacteria, with a focus on the global issue of antimicrobial resistance.
Combatting antimicrobial resistance: alternative technologies?
Laura Bowater
The problem of antimicrobial resistance is one of the most significant threats facing global health, wellbeing and food security. As of June 2019, only 42 new antibiotics, with the potential to treat serious bacterial infections, are in clinical development and only one in five is likely to gain approval. The seminal O’Neill report on antimicrobial resistance, released in 2016, emphasised the need to develop new antibiotics but gave a compelling argument for the need to develop new solutions and treatments to replace our antibiotic reliance.
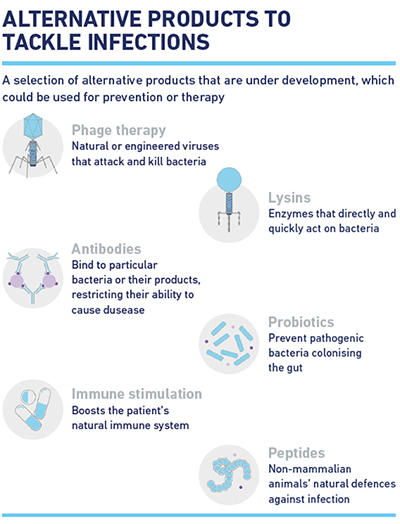
So, what are these alternative solutions? Some are well established pharmaceutical products such as immunotherapies that are effectively treating other diseases and may be able to treat or prevent bacterial infections. Others are new therapies. For example, lysins are hydrolytic enzymes derived from bacteriophages that target and disrupt bacterial cell walls and are currently being trialled against Gram-positive and recently Gram-negative pathogens. Other solutions include virulence inhibitors, such as products that disrupt the cooperative social behaviour of bacteria, alleviating their pathogenic threat to patients, or products that replace harmful bacteria with ‘healthy’ bacteria. It is reassuring to see that there is still a keen interest in hunting down novel bacterial targets. One example is oligonucleotide transcription factor decoys (TFDs), molecules that resemble the binding sites for essential bacterial transcription factors. When delivered on nanoparticles into bacterial cells they act as decoys, preventing expression of the genes needed for the bacteria to survive and cause infection.
The good news is we currently have 29 alternative candidates in clinical development compared to the 42 new antibiotics in current pipelines. The bad news is most non-traditional products are only active against a limited range of pathogens and the establishment of regulatory guidelines for their approval is still in its infancy. Nevertheless, the hunt continues…
Further reading
The Pew Trust. Assessment of nontraditional products in development to combat bacterial infections; 2019. https://www.pewtrusts.org/pt/research-and-analysis/issue-briefs/ 2019/09/assessment-of-nontraditional-products-in-development-to-combat-bacterial-infections [accessed 30 January 2020].
Köhler T, Perron GG, Buckling A, van Delden C. Quorum sensing inhibition selects for virulence and cooperation in Pseudomonas aeruginosa. PLoS Pathog 2010;6: e1000883. DOI:10.1371/journal.ppat.1000883
Procarta Biosystems. https://www.procartabio.com [accessed 30 January 2020].
AMR Review. Tackling drug-resistant infections globally: final report and recommendations. https://amr-review.org/sites/default/files/ 160518_Final%20paper_with%20cover.pdf [accessed 30 January 2020].
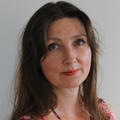
Laura Bowater
University of East Anglia, Norwich Research Park, Norwich, Norfolk NR4 7TJ, UK
[email protected]
@ProfLauraBow
Laura Bowater is currently Academic Director for Innovation and a Professor of Microbiology Education and Engagement. She was previously Associate Dean for Enterprise and Engagement in the Faculty of Medicine and Health. Laura graduated from the University of St Andrews with a BSc in Biochemistry with Microbiology then moved to the University of Dundee to study for an MSc and PhD in Microbiology. After a four-year career break to raise her family, she took up a postdoctoral post at the John Innes Centre, attained an MA in Education from the University of East Anglia, worked for the Open University and then moved to the University of East Anglia to focus on teaching, science communication and public engagement.
Why does microbiology matter?
Because almost every aspect of life as we know it relies on the intricate relationship we have with the microbial world.
In your opinion, which areas of research are likely to have the greatest impact on tackling antimicrobial resistance in the future?
There are a lot of research areas to choose from. In my mind, I think it is vital that we begin to refill our pharmaceutical cupboards that are starting to look uncomfortably bare. Therefore, searching for new antimicrobial compounds would be a top priority for me.
The world of biofilms
Thomas James O’Brien and Martin Welch
Bad news folks; ever since modern microbiology emerged as a distinct discipline in the latter part of the 19th century, it looks like we’ve been studying the wrong thing. Those colonies on your agar plates or flasks of liquid culture in your orbital shaker are about as physiologically relevant as the medium which you’re growing them in. That’s because it turns out that most bacteria spend most of their time doing things differently – they live out their lives either stuck to one another or attached to the debris and organic matter floating around them. This realisation is somewhat ironic, especially given that microbial communities attached to solid surfaces (teeth) were among the very first things to be described by Antonie van Leeuwenhoek using his prototype microscopes in the 17th century. However, it wasn’t until the 1930s that Arthur T. Henrici pushed the point home by commenting: “it is quite evident that for the most part water bacteria are not free-floating organisms but grow attached upon submerged surfaces”. The expression ‘biofilm’ was born shortly afterwards. If you think that this would have catalysed rapid progress, you’d be wrong. Although most ‘environmental microbiologists’ had been wittingly studying biofilms for decades, the field did not emerge from its lag phase within mainstream microbiology until the mid-1980s. The most likely reasons for this are two-fold. First, scientists hate mess. We like to work with pure axenic cultures grown from isolated colonies, cultured in defined laboratory media. Why would we possibly want to add bits of floating detritus (as biofilm substrata) to these pure, defined cultures? Second, it wasn’t until the mid-1980s that the link between biofilms and chronic infection was first proposed. But that was a game changer…
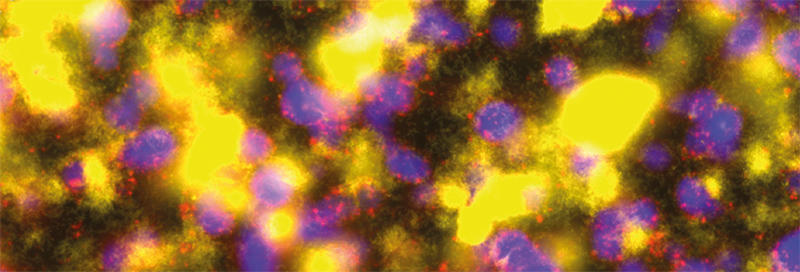
The formation of biofilms
It turns out that there is a loose, but nevertheless compelling, link between growth mode and infection severity/type. Acute infections are generally associated with the planktonic growth mode, whereas chronic infections are generally associated with biofilm formation. By definition, chronic infections (such as those associated with cystic fibrosis or endocarditis) are poorly resolved by the immune system and/or antibiotic intervention. This is partly because biofilms are poorly permeable to some antibiotics, and partly because the slow-growing cells in the biofilm’s centre are less susceptible to these agents. More insidiously, the high density and close proximity of micro-organisms in biofilm communities promotes the horizontal transfer of antimicrobial resistance (AMR) determinants, particularly through contact-dependent mechanisms such as conjugation. These realisations have driven an explosion in biofilm research over the last two decades. Indeed, a simple PubMed search reveals that the number of articles mentioning the word ‘biofilm’ remains on an exponentially increasing trajectory, with well over 5,000 publications last year alone. But we’re getting a bit ahead of ourselves here – what exactly are biofilms and why should we care about them?
The physiology of biofilms
Biofilms are essentially quasi two-dimensional assemblages comprising one or more microbial species, held together by a self-produced extracellular polymeric ‘glue’ (termed matrix). As outlined above, such aggregates may be anchored to a solid (or semi-solid) substratum, or they may form free-floating assemblies called flocs. Environmental biofilms (and some clinical biofilms too) are more often than not polymicrobial (i.e. containing more than one species) and can reach cell densities as high as 1011 cells (g wet weight)-1. Living cheek by jowl in the centre of such a densely packed community does pose several problems, not least the fact that your cousins at the surface will quickly consume all the oxygen and nutrients before you get a look-in. Therefore, it is not surprising that biofilm physiology changes rapidly with increasing depth and the individual cells comprising the structure are exceptionally heterogenous. The self-organised structural heterogeneity in biofilms is abundantly clear in laser scanning confocal microscopy studies, which reveal that biofilms are comprised of spatially separated heterogenous sub-populations, called microcolonies. The complex spatial organisation and accompanying chemical gradients strongly promote genetic diversity within the population, as no single species is perfectly adapted for every available environmental niche.
Biofilms and AMR
As mentioned earlier, biofilms are very much at the forefront of microbiological research these days, because of their role in AMR. In addition to their phenotypic and genetic heterogeneity, another reason biofilms remain resistant to antimicrobial agents is because the polysaccharide matrix itself forms a physical protective barrier against environmental stressors. The matrix also limits the diffusion of extracellular molecules away from the population, allowing for the sharing of public goods and a division of metabolic labour.
Thankfully, biofilms are not always bad news. As far back as 1860, engineers were inadvertently using the biofilms associated with sand particles to clean up wastewater in sewage treatment plants. In a similar vein, biofilms play a key role in the bioremediation of industrial effluents and environmental pollutants, including dairy industry waste and marine oil spills, respectively. More recently, this field has seen a resurgence of interest, with anode-associated biofilms used in microbial fuel cells and several high-value bioproducts being made by exploiting the unique catalytic potential of biofilms. Ongoing research into the complex world of biofilms is aimed at developing novel treatments for the removal and prevention of biofilm growth in problematic scenarios, as well as unlocking and harnessing their full potential in industrial applications. But don’t hold your breath; it is unlikely that there will be a universal Achilles heel to target. Biofilms have been around for billions of years (try Googling ‘stromatolites’) and, as pointed out earlier, they represent the default growth mode for most bacteria most of the time. Just as many roads lead to Rome, it is highly likely that there are many ways to make a biofilm.
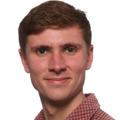
Thomas James O’Brien
Department of Biochemistry, Hopkins Building, Tennis Court Road, University of Cambridge, Cambridge CB2 1QW, UK
Thomas James O’Brien is a PhD student and Research Assistant in the Department of Biochemistry at the University of Cambridge. His research aims to develop an in vitro polymicrobial model to recapitulate and study the complex microbial communities associated with cystic fibrosis airway infections. He is particularly interested in interspecies communication and microbial ecology at the community-wide level. He has been a member of the Microbiology Society for three years (since the start of his PhD) and has found the support given by the Society to be an invaluable resource throughout his studies.
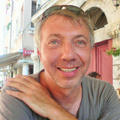
Martin Welch
Department of Biochemistry, Hopkins Building, Tennis Court Road, University of Cambridge, Cambridge CB2 1QW, UK
Martin Welch was born near Cambridge, and studied biochemistry at Oxford. He studied for his PhD at the Weizmann Institute of Science in Israel, and followed this with a short postdoc in Toulouse where he learned how to solve x-ray crystal structures. Now back in Cambridge, his laboratory currently focuses on understanding the link between bacterial metabolism and virulence/lifestyle choices. In his spare time, Martin enjoys cooking and horse riding. He is also passionately interested in local history and natural history.
Why does microbiology matter?
Thomas: Micro-organisms are the most versatile and abundant life-form on the planet, inhabiting every known environmental niche – no matter how treacherous or desolate. Not only are they truly fascinating organisms to study at the molecular level, but they play a focal role in undertaking and modulating natural biochemical processes that occur across the entirety of the Earth’s surfaces and within our own bodies, especially as micro-organisms outnumber our own cells 10 to 1! Whether the action of these micro-organisms is positive or detrimental, fully understanding how they work and what regulates these will allow us to harness and adapt the power of micro-organisms for our own benefit and better tackle infectious disease, especially with the worrying rise of antimicrobial resistance.
How did you enter this field?
Thomas: I first became interested in microbiology when studying ‘general biology’ at college. I was fascinated by the ability of microscopic, and seemingly inconsequential, organisms to eventually adapt to and overcome any therapeutic or environmental challenge they may face – especially in the context of infectious disease. As such, I chose to enrol in a microbiology course for both my undergraduate and master’s studies at the University of Leeds. Here, my fascination grew as I began to learn more and more about the truly endless world of microbiology, cementing my desire to enter the world of research at the academic level. Hence, I pursued several lab-based projects throughout my studies in order to prepare myself for undertaking a PhD and hopefully I will be able to continue working within this amazingly diverse field as my studies begin to draw to a close.
Bacteria in industry: microbial bio-factories for a sustainable future
Anna Trego and Vincent O’Flaherty
Often, bacteria carry a bit of a bad rap – being commonly associated with spoiled food and infectious disease, when in fact, fewer than 1% of the known microbial species on the planet are pathogenic. Moreover, we rely on many different bacteria, archaea, fungi, yeasts and moulds for the biochemical conversions that are part of their natural genetic machinery. Indeed, microbial cells are essentially miniature bio-factories, producing a wide range of useful products. Evidence suggests that over 6,000 years ago, micro-organisms were used by humans to make bread, wine, beer and cheeses. People didn’t understand the fermentative processes which they were exploiting, and fermentation wasn’t linked to microbial metabolism until 1857 when Louis Pasteur discovered that yeast cells were responsible for the conversion from sugar to alcohol.
Microbial potential
Since then, our use of microbial metabolism has rapidly expanded and has completely revolutionised food production, healthcare, chemical production and waste treatment. Advancements in genetic engineering in the 1960s and 1970s saw the rise of numerous medical biotech companies using microbial cells to produce helpful biological substances – an industry estimated to be worth over 700 billion by 2025. These companies often use genetic engineering to patent bacterial super-strains which quickly pump out a wide range of specialised enzymes, hormones, probiotics, antibiotics and vaccines, providing treatments for a number of medical conditions such as diabetes, tuberculosis (TB), AIDS, various cancers and systemic lupus erythematosus (SLE). The outlook and potential for such industries is boundless. Indeed, it is now proposed that Earth may be home to as many as one trillion different species of microbes – 99.99% of them are yet to be discovered, meaning that the untapped microbial metabolic potential is enormous.
While the past few decades have unleashed a flood of biotechnological advancements with a strong industrial focus on combating disease, there are other challenges facing humanity – specifically, global population increase coupled with climate change. Increased pressures in food production are to be expected, waste generation will increase, water will become scarcer and energy consumption will increase. We are going to need innovative solutions to address such challenges. Could sustainable solutions be hidden in the genetic makeup of micro-organisms?
Microbes for sustainable food production
Traditionally, bacteria and yeasts are important for the direct manufacture of a variety of food products including chocolate, coffee, wine, beer, bread, meat products such as sausages, dairy products and pickled goods such as sauerkraut, pickles and olives. Recently, however, a somewhat controversial concept has been revisited for the sustainable production of protein with a low environmental footprint. Traditional plant- and animal-based protein sources are quickly becoming unsustainable and scientists are turning to bacteria, algae, yeasts and fungi for the production of protein-rich feed or food additives as a more sustainable option. This microbial protein (MP) can be developed for human consumption, or as a microbial alternative for the protein supplements added to agricultural feed. For example, FeedKind®, developed in Norway and for sale in the EU, is a fish feed (for fish farming) produced by Methylococcus capsulatus from natural gas. In comparison with traditional soy- and fishmeal-based feeds, its production has much lower land and water requirements. Alternatively, for human consumption, fungal production of MP has been successful. QuornTM, a mycoprotein meat alternative, produced by Fusarium venenatum, has expanded into the global market and expects to be a billion-dollar business by 2027. The success of these emerging MP-based industries will likely generate growing interest in sustainable, microbial food additives.
Microbes for sustainable wastewater treatment, resource recovery and energy production
Microbiology and microbial industrial technologies are key for environmental sustainability. In natural environments, such as soils and oceans, micro-organisms are the major drivers of biogeochemical and elemental cycles that maintain the biosphere. Their extraordinary metabolic diversity is also exploited in environmental engineering systems, such as wastewater treatment plants. Microbiology will play a key role in how society achieves the UN Sustainable Development Goals. A growing human population, particularly in urban areas, will produce more wastewater, which requires treatment before being safely discharged into the environment – but clean water and sanitation are still not available to millions. Generally, wastewater treatment involves a biological component – where active micro-organisms metabolise and remove organic pollutants. There are several approaches to this. Traditionally, aerobic treatment facilities were implemented and have been highly successful all over the world, but they have important drawbacks associated with cost, energy and scalability. By comparison, the alternative anaerobic microbial treatment process is low-cost, more energy efficient and can meet all criteria for water protection. Furthermore, anaerobic treatment, which is underpinned by a complex consortium of micro-organisms from various trophic groups, can completely convert complex organic compounds into a combustible biogas in the form of methane – a renewable energy source. This technology, which took off in the 1970s, has expanded from lab-scale research to full-scale industrialised bioreactors all over the world. According to a recent report, the global anaerobic digestion industry is projected to grow to over $16 billion by 2026. But it’s no longer just about securing water sources and generating energy; there is an increasing focus among researchers and industry alike, in not just removing nutrients from wastewater, but in using bacteria and archaea to recover valuable sources of nitrogen and phosphorus – both principal components in fertilisers.
Outlook
Not only do microbes provide the foundation for many billion-dollar businesses spanning multiple industrial sectors, but they also likely contain the genetic power to provide us sustainable solutions to the growing pressure on Earth’s finite resources. As Louis Pasteur said so many years ago, microbes really will have the last word.
Further reading
Leroy F, De Vuyst L. Lactic acid bacteria as functional starter cultures for the food fermentation industry. Trends Food Sci Technol 2004; 15:67–78. DOI:10.1016/j.tifs.2003.09.004.
Matassa S, Boon N, Pikaar I, Verstraete W. Microbial protein: future sustainable food supply route with low environmental footprint. Microb Biotechnol 2016;9:568–575. DOI:10.1111/1751-7915.12369
McKeown RM, Hughes D, Collins G, Mahony T, O’Flaherty V. Low-temperature anaerobic digestion for wastewater treatment. Curr Opin Biotechnol 2012;23:444–451. DOI: 10.1016/j.copbio.2011.11.025
O’Flaherty V, Collins G, Mahony T. The microbiology and biochemistry of anaerobic bioreactors with relevance to domestic sewage treatment. Rev Environ Sci Bio/Technology 2006;5:39–55. DOI: 10.1007/s11157-005-5478-8
van Lier JB, van der Zee FP, Frijters CTMJ, Ersahin ME. Celebrating 40 years anaerobic sludge bed reactors for industrial wastewater treatment. Rev Environ Sci Bio/Technology 2015;14:681–702. DOI:10.1007/s11157-015-9375-5
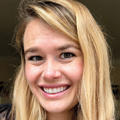
Anna Trego
National University of Ireland (NUI), Galway, University Road, Galway H91 TK33, Ireland
Anna Trego, a native Californian, graduated from the University of Arizona with a bachelors degree in Environmental Science, before serving two years with Teach for America as a chemistry and physics teacher. She then completed her MSc in Sustainable Resource Management at the University of Limerick and the NUI Galway. She recently completed her PhD at NUI Galway – studying microbial community development of biofilms used for biological wastewater treatment. She now works with Professor Vincent O’Flaherty as a postdoctoral researcher in microbial ecology.
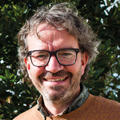
Vincent O’Flaherty
National University of Ireland (NUI), Galway, University Road, Galway H91 TK33, Ireland
Vincent O’Flaherty is Professor of Microbiology at the National University of Ireland, Galway. His expertise is in the field of microbial ecology and bioprocess research. To date, three start-up companies have been formed arising from research in his laboratory. He teaches and learns about microbiology and environmental biotechnology with students at all levels.
Why does microbiology matter?
Anna: Microbiology matters almost inherently. Not only are our bodies filled with microbes – indeed we are just now starting to uncover how important the human gut microbiome is to our overall health and wellbeing – but as far as we know, micro-organisms are the most evolutionarily ancient life forms on the planet. They can be found in even the most inconceivable of environments: hot, cold, salty, anoxic, acidic, you name it, there’s a microbe that can make it work. Understanding the structure and function of micro-organisms and microbial communities can help unlock the secrets of the evolution of life on our planet, can help us live healthier, longer lives and can potentially provide the basis for sustainable solutions to increased pressure on natural resources.
On a typical day (or week) in your job what do you do?
Anna: I am a Postdoctoral Researcher at the National University of Ireland, Galway. On a typical day I can be found in the microbial ecology lab where, together with my colleagues, I study mixed microbial communities, how they respond to changing environmental conditions, how they interact with one another, and how we can apply their natural functions for the sustainable production of energy, biopolymers or valuable industrial chemicals. One of the most challenging parts of our work is that the micro-organisms that we study are anaerobic, meaning that oxygen is toxic to them. We have to create special, completely enclosed environments with no oxygen in order to study them.
Images: Biofilm Battleground: Confocal laser scanning microscopy image taken of a co-culture of Pseudomonas aeruginosa PAO1 (yellow cells) and P. aeruginosa PAO4 (blue cells). Propidium iodide labelling of compromised cells (red spots) reveals that PAO1 kills off PAO4 cells present within the mixed species biofilm. Dr Nuno Miguel Oliveira, Department of Applied Mathematics and Theoretical Physics, University of Cambridge.
Review on Antimicrobial Resistance.
Find out more about 'Understanding bacteria and the challenges in microbiology' in our digital content hub.
-
Understanding bacteria and the challenges in microbiology
There are approximately 10 times as many bacterial cells as human cells in the human body. They are found in every habitat on Earth, therefore in an era where antimicrobial resistance has become a global issue, developing novel antimicrobial strategies, discovering the world of biofilms and understanding bacteria in industry is important to the world of microbiology.
Image: Judith Armitage.