SLIC: a novel technology to rapidly detect bacteria
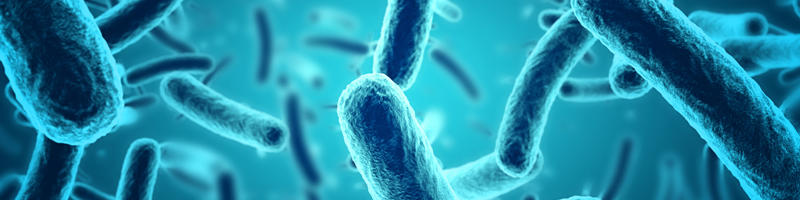
The Microbiology Society is undertaking a project entitled A Sustainable Future as part of our 75th Anniversary, which aims to highlight the Sustainable Development Goals (SDGs) to our members and empower them to use their research to evidence and impact the goals. Earlier this year, we put a call out to our members to submit case studies in the following three areas: antimicrobial resistance, soil health and the circular economy.
This case study is written by Dr Robert Hammond, who is an Industrial Research Fellow at the University of St Andrews, and a member of the Microbiology Society. It focuses on antimicrobial resistance; a naturally occurring process, whereby micro-organisms (bacteria, viruses, fungi and parasites) can change and adapt over time, either by modifying the target of the antimicrobial, or by developing and exchanging resistance genes.
Scattered Light Integrating Collector (SLIC) is an exciting novel technology with a plethora of potential uses. As microbiologists, we at the University of St Andrews have chosen to use it to study microbial growth and change under experimental conditions in order to develop a point-of-care diagnostic tool.
SLIC is a rapid phenotypic test that can detect microbial growth in less than 10 minutes from a clinical sample and the drug resistance profile for the infecting organism in less than 30 minutes. SLIC’s revolutionary sensitivity, which allows the detection of around 25 bacteria per 2ml of liquid, means that it can address some of the most pressing antimicrobial resistance (AMR) problems the world is currently facing. Because bacteria and fungi divide constantly, under the correct circumstances, small changes in their populations can be detected. This incremental increase could be vital in understanding the effects of antibiotics known to be affected by inoculum size – namely beta lactams. It also could mean the difference between sending a patient home with no treatment, the wrong treatment or an inappropriate treatment when they present with a nascent urinary tract infection (UTI), that was undetectable or below local thresholds for therapy.
The more we use SLIC, the more we discover that it has capabilities beyond those that were originally designed. Excitingly, we discovered that the technology was able to distinguish antibiotic action in real time.
Antimicrobials are either defined as bactericidal (kills bacteria) or bacteriostatic (prevents the growth of bacteria). Bactericidal antimicrobials are sub-classified into lytic and non-lytic. This is an important definition for SLIC, as it can determine the difference between cell death and cell lysis. With these broad characterisations, we determined the different modes of action for various antibiotics against various organisms. Some of our findings are very interesting and have direct implications for human health. We found that antibiotics that bind to penicillin-binding proteins (PBPs), specifically PBP 2 & 3 in Enterobacteriacea, cause the cell to elongate before it is killed. This is not new, but what is novel, is the way cell death occurs after the cells elongate to a certain size. We have also shown that E. coli, treated with a cephalosporin, will elongate for less than three hours until a critical mechanical threshold is reached after which the cells will dissociate and lyse. This appears to us in the SLIC readout as growth above the control, then a sharp decline in signal as the cells lyse. However, the rapidity of the cell lysis and dissociation was surprising and can account for the occasionally unusual activity of cephalosporins in patients.
Beyond this example, SLIC has also revealed the importance of dosing in the case of drugs such as carbapenems. We have shown a dose-dependent killing effect that is mathematically derived from the fold-increase or decrease, above or below the minimum inhibitory concentration (MIC) for a given organism. This insight has meant that we can use a dose of antibiotic, be confident it will be effective at a given concentration and know when that effect will occur.
SLIC was originally designed with low and middle-income countries (LMIC) in mind and we have not lost sight of that vision. The current prototypes are cheap to manufacture, require very few consumables, run on less than 12 volts and are very easy to use. The consumables we use have a very low cost associated per run. Each run consists of five separate tests for bacterial presence and susceptibility, therefore each test costs very little. All the consumables we currently use are off-the-shelf and are easy to obtain globally. We have loaned SLIC machines to various healthcare facilities and have had positive feedback from all partners in terms of data generated and ease of use. SLIC relies upon basic fluid handling techniques all qualified laboratory workers are well versed in. The technology is also robust and rugged. We have shipped machines internationally with no problems and have found that, even in non-ideal environments, the machines work admirably.
All of this is to say that we have created a machine that can be manufactured at low cost and used in all environments, even with intermittent power. We believe SLIC is the answer to the question of how we are going to turn the tide on AMR in both the developed and the developing world.
About the author
Dr Robert Hammond is an Industrial Research Fellow at the University of St Andrews, and a member of the Microbiology Society. More information about his work is available here.