The PET-biodegrading potential of the Plastisphere
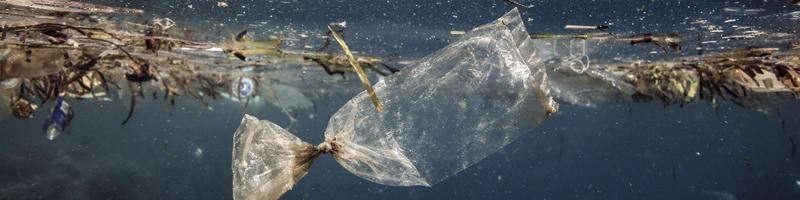
The Microbiology Society is undertaking a project entitled A Sustainable Future as part of our 75th Anniversary, which aims to highlight the Sustainable Development Goals (SDGs) to our members and empower them to use their research to evidence and impact the goals. Earlier this year, we put a call out to our members to submit case studies in the following three areas: antimicrobial resistance, soil health and the circular economy.
This case study is written by Dr Robyn Wright, who is a Postdoctoral Research Fellow at Dalhousie University, Canada, and a member of the Microbiology Society. It focuses on the Circular Economy; an alternative to a traditional linear economy (make, use, dispose), in which we keep resources in use for as long as possible, extract the maximum value from them while in use, then recover and regenerate products and materials at the end of each service life.
Plastics now pollute marine environments across the globe and, on entering these environments, plastics are rapidly colonised by a diverse community of micro-organisms termed the Plastisphere. [1]. A number of studies have claimed the presence of plastic-biodegrading organisms within the Plastisphere, although this has generally been without mechanistic confirmation. One remarkable exception to this is in the degradation of the common packaging plastic, polyethylene terephthalate (PET), where a terrestrial bacterium, Ideonella sakaiensis – isolated from outside a plastic bottle factory in Japan – has been found to produce a PETase (PET hydrolase), an enzyme that exhibits higher hydrolytic activity on PET than on other substrates tested. [2] This discovery has led to other researchers finding a number of PETase homologs in marine metagenomes, [3] although no marine microbes with this ability have so far been isolated. With an estimated 100 million metric tons of plastics now in the oceans, [4] determining whether this can be efficiently biodegraded is of utmost importance, as well as the identification of enzymes for plastic degradation potentially leading to important applications in the biological recycling of plastics [5].
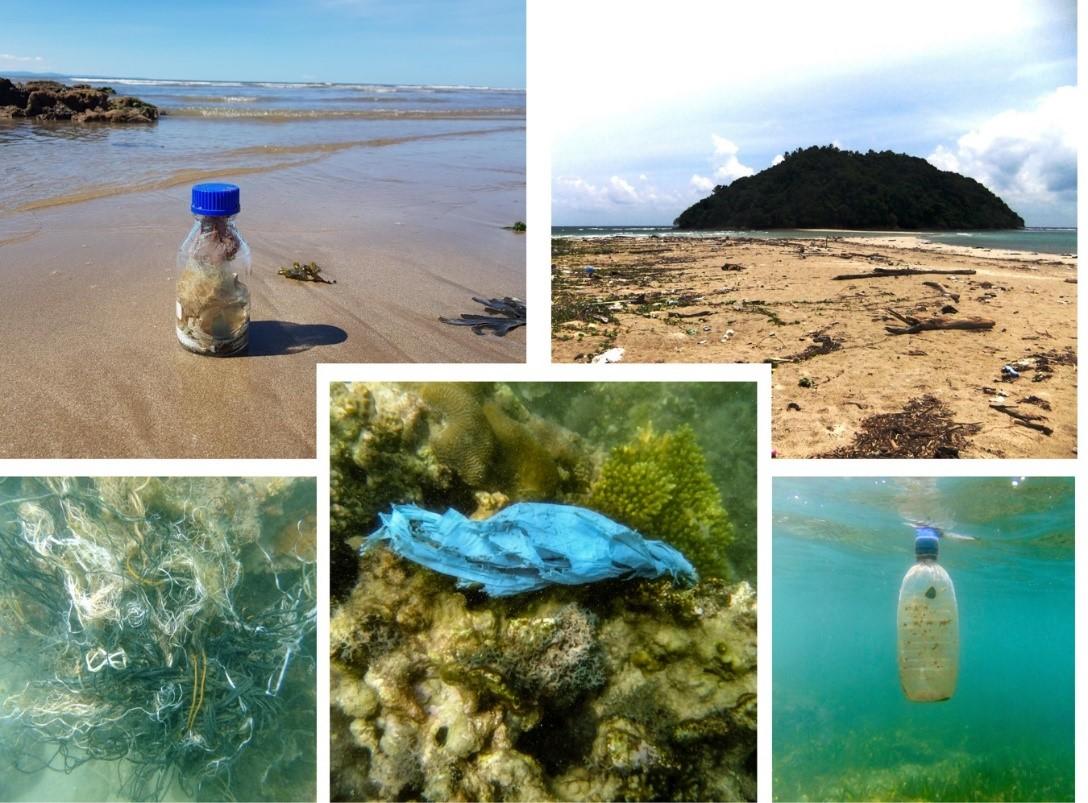
Clockwise from left: Collecting plastics in Porthcawl, Wales; plastics on the beach near Kudat, Borneo; a plastic bottle floating in the ocean near Kudat, Borneo; a plastic bag on a coral reef near Semporna, Borneo; fishing ropes tangled on a coral reef near Semporna, Borneo.
During my PhD research, I isolated two marine microbes that could grow well on a PET degradation and production intermediate (bis (2-hydroxy ethyl) terephthalate; BHET). I found that the genomes of both isolates contained potential PETase homologs, and I characterised their ability to biodegrade PET using a combined proteomic and metabolomic approach. Although work on this is ongoing, this enabled me to confirm that PET degradation intermediates were produced by both bacteria, and that only one of the isolates appears to use a pathway similar to that previously identified in I. sakaiensis. Intriguingly, the other isolate exhibited far higher oxidation of the PET surface, which could mean that this isolate is using enzymes that share little homology with the previously identified PETases, or even that it uses a novel pathway for PET degradation, although this remains to be determined.
Of course, in nature the degradation of compounds is much more complex than just whether a member of the microbial community is capable of it. In some cases, the degradation of a compound by one species may lead to a build-up of a potentially toxic intermediate, for example the build-up of phthalic acid that we found during plastic additive degradation [6] (very similar to the terephthalic acid produced during PET degradation). This can lead to interactions between microbial community members, where the metabolites produced by one species are used by another species, [7] thus reducing the toxicity to the first species. These interactions between species, however, can also be antagonistic, for example in the case of the marine degradation of some polysaccharides, where one species broadcasts degrading enzymes and a secondary consumer uses the products of this degradation and actually eventually inhibits the particle degradation and therefore growth of both species. [8]
Natural microbial communities go through distinct stages of succession, characterised by: (i) an initial colonisation phase; (ii) a selection phase, characterised by an enrichment of organisms that are capable of polymer degradation; and (iii) a succession phase, where efficient degraders are overtaken by cheaters, cross-feeders and grazers. [9, 10] When I examined microbial community succession on PET (using amplicon sequencing of the 16S rRNA gene), I found that the same was true here, but metabolomic analyses didn’t identify any degradation intermediates, which could be attributed to the rapid depletion of intermediates by other community members. If we wish to exploit microbial communities for the degradation of PET then it will be important to more thoroughly characterise the dynamics of PET degradation within the Plastisphere and to assess the metabolic capacity of each community member in detail. The use of synthetic communities could further reduce antagonistic interactions and remove the natural element of microbial community succession while also avoiding the build-up of toxic intermediates.
While the work I’ve described here is ongoing, further studies into the metabolic capacity of the Plastisphere will hopefully lead to more definitive answers on the biodegrading potential of the Plastisphere. There is also the possibility that this could yield new methods for the biological recycling of PET, either by isolates or communities.
References
1. Amaral-Zettler LA, Zettler ER, Mincer TJ. Ecology of the plastisphere. Nat Rev Microbiol 2020;18:139–151.
2. Yoshida S, Hiraga K, Takehana T, Taniguchi I, Yamaji H, et al. A bacterium that degrades and assimilates poly(ethylene terephthalate). Science (80- ) 2016;351:1196–1199.
3. Danso D, Zimmermann, Schmeisser C, Chow J, Zimmermann W, et al. New insights into the function and global distribution of polyethylene terephthalate (PET) degrading bacteria and enzymes in marine and terrestrial metagenomes. Appl Environ Microbiol 2018;84:e02773-17.
4. Jambeck J, Geyer R, Wilcox C, Siegler T, Perryman M, et al. Plastic waste inputs from land into the ocean. Science (80- ) 2015;347:768–771.
5. Salvador de Lara M, Abdulmutalib U, Gonzalez J, Kim J, Smith AA, et al. Genes for a circular and sustainable bio-PET economy. Genes (Basel) 2019;10:1–15.
6. Wright RJ, Bosch R, Gibson MI, Christie-Oleza JA. Plasticizer degradation by marine bacterial isolates: a proteogenomic and metabolomic characterization. Environ Sci Technol 2020;54:2244–2256.
7. Cavaliere M, Feng S, Soyer O, Jiminez J. Cooperation in microbial communities and their biotechnological applications. Environ Microbiol 2017;19:2949–2963.
8. Enke TN, Leventhal GE, Metzger M, Saavedra JT, Cordero OX. Microscale ecology regulates particulate organic matter turnover in model marine microbial communities. Nat Commun 2018;9:1–8.
9. Datta MS, Sliwerska E, Gore J, Polz MF, Cordero OX. Microbial interactions lead to rapid micro-scale successions on model marine particles. Nat Commun 2016;7:1–7.
10. Wright RJ, Gibson MI, Christie-Oleza JA. Understanding microbial community dynamics to improve optimal microbiome selection. Microbiome 2019;7:1–14.
About the author
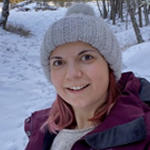
Dr Robyn Wright is currently a Postdoctoral Research Fellow in the Langille Lab at Dalhousie University, Nova Scotia, Canada. The research described here was carried out predominantly during Robyn’s BBSRC-funded PhD (through the Midlands Integrative Biosciences Training Partnership) at the University of Warwick, UK, working with Dr Joseph Christie-Oleza (now at the University of the Balearic Islands, Spain) and Prof Matt Gibson (University of Warwick). More information about her work is available here.